2024 Summer Undergraduate Research Highlights
From missions to the Moon & Mars, to lake sediments, to climate modeling, many undergraduate students worked on groundbreaking research in DEEPS this summer break. This year, we are excited to showcase these diverse research projects through a special combination of art, writing, and photography developed by Claire Xu ‘27.
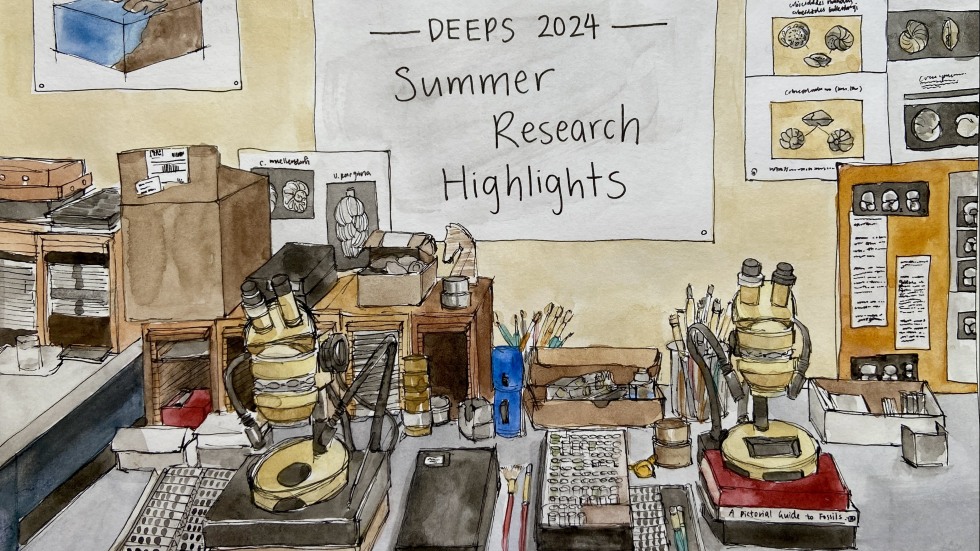
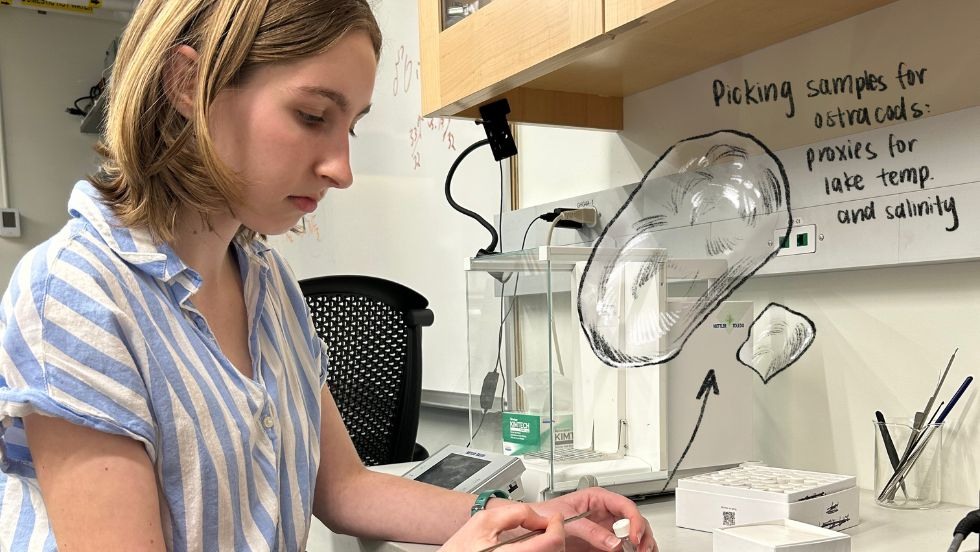
Anna Dubey: "I am analyzing Western U.S. ostracod fossils from Silver Lake, historically Glacial Lake Mojave, to assess the lake’s conditions across the last deglaciation." (Advisor: Dan Ibarra)
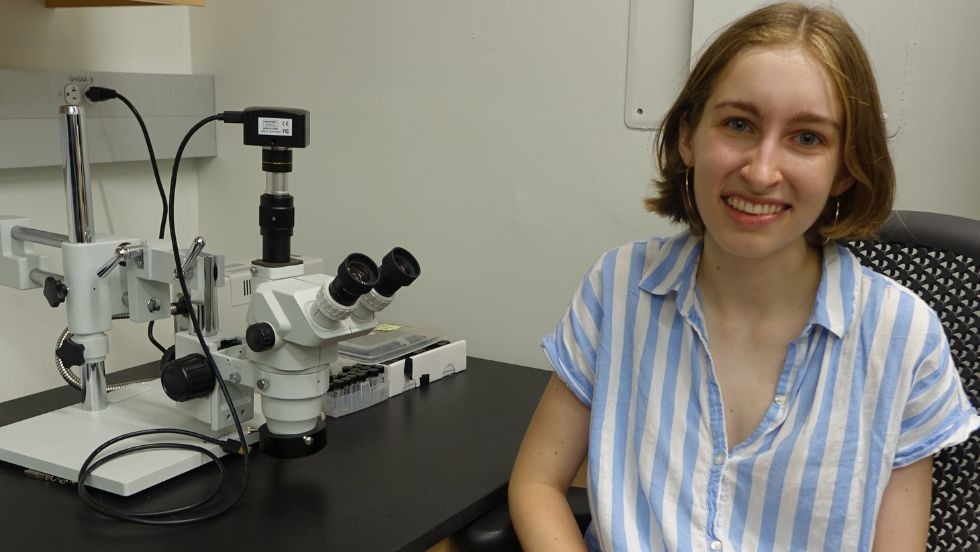
Anna Dubey: "I am analyzing Western U.S. ostracod fossils from Silver Lake, historically Glacial Lake Mojave, to assess the lake’s conditions across the last deglaciation." (Advisor: Dan Ibarra)
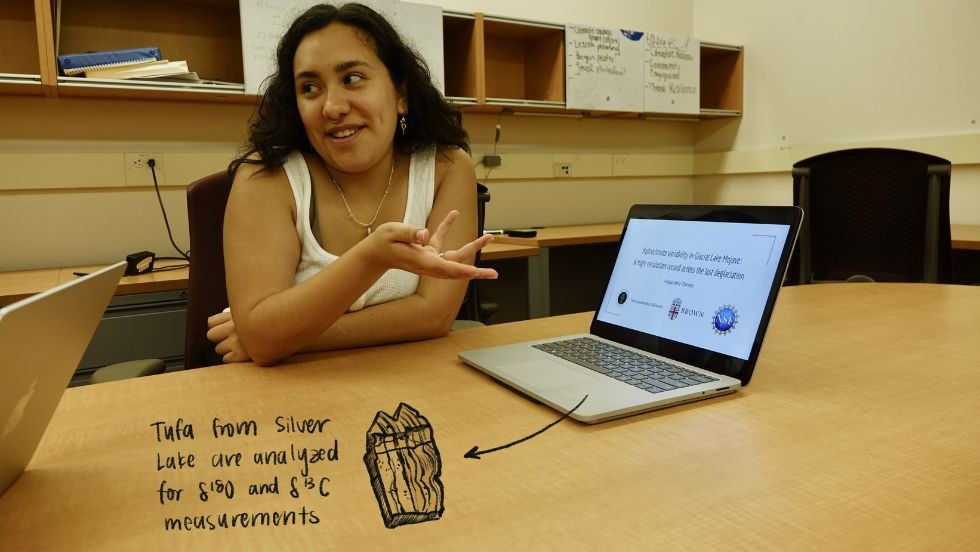
Alejandra Torres: “I am creating a time series using stable isotope analysis of tufa records from Glacial Lake Mojave (also known as Silver Lake, located near modern day Los Angeles, CA) to assess changes in lake conditions across the last deglacial period." (Advisor: Dan Ibarra, 2024 DEEPS-Leadership Alliance REU student from Dallas College)
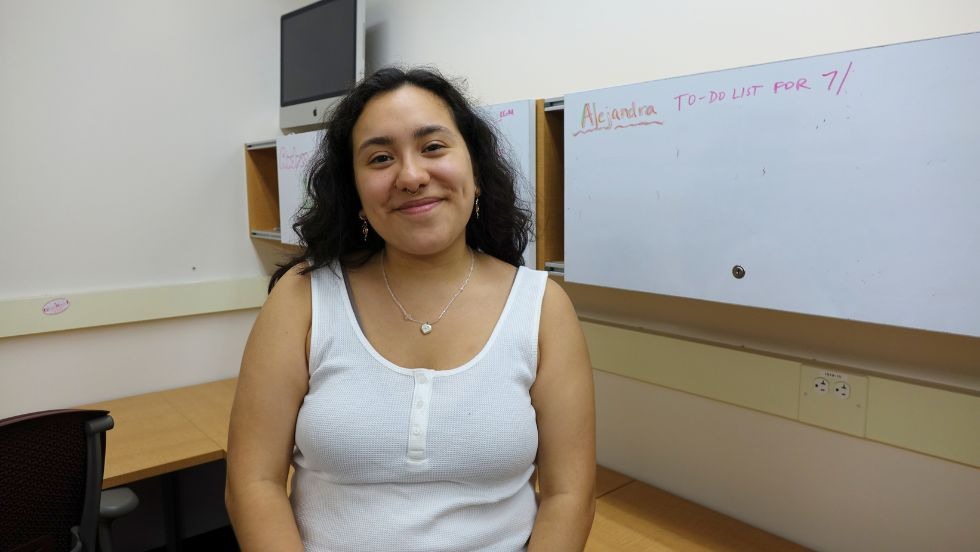
Alejandra Torres: “I am creating a time series using stable isotope analysis of tufa records from Glacial Lake Mojave (also known as Silver Lake, located near modern day Los Angeles, CA) to assess changes in lake conditions across the last deglacial period." (Advisor: Dan Ibarra, 2024 DEEPS-Leadership Alliance REU student from Dallas University)
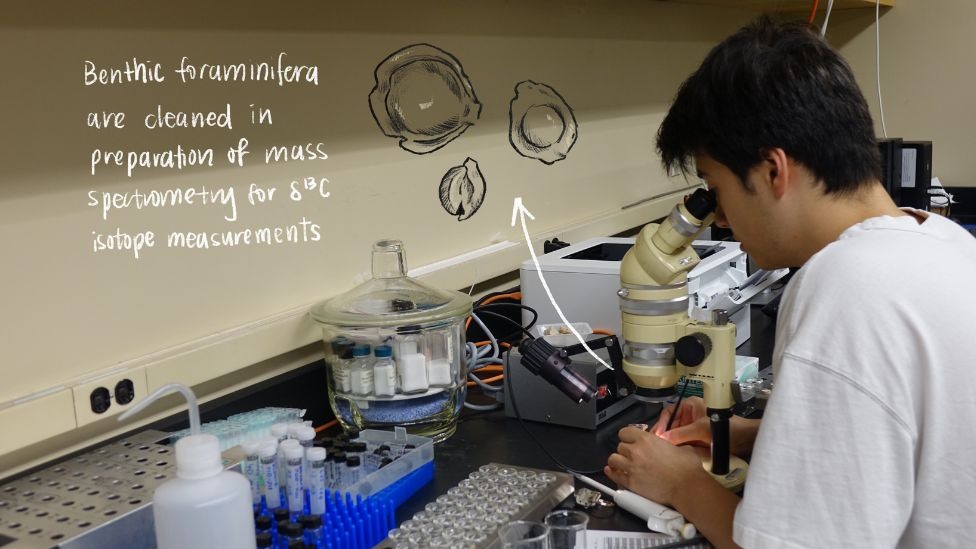
Galen Gibb: “I am using benthic foraminiferal fossils of the Milioda order to uncover the extent of methane hydrate dissociation and subsequent methane release in the Indian Ocean over the past 1.4 million years." (Advisor: Steve Clemens)
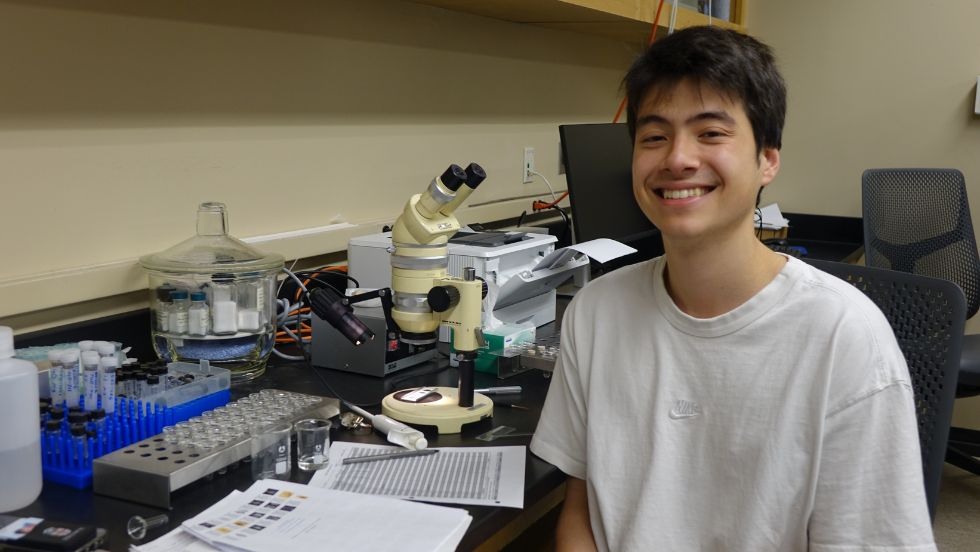
Galen Gibb: “I am using benthic foraminiferal fossils of the Milioda order to uncover the extent of methane hydrate dissociation and subsequent methane release in the Indian Ocean over the past 1.4 million years." (Advisor: Steve Clemens)
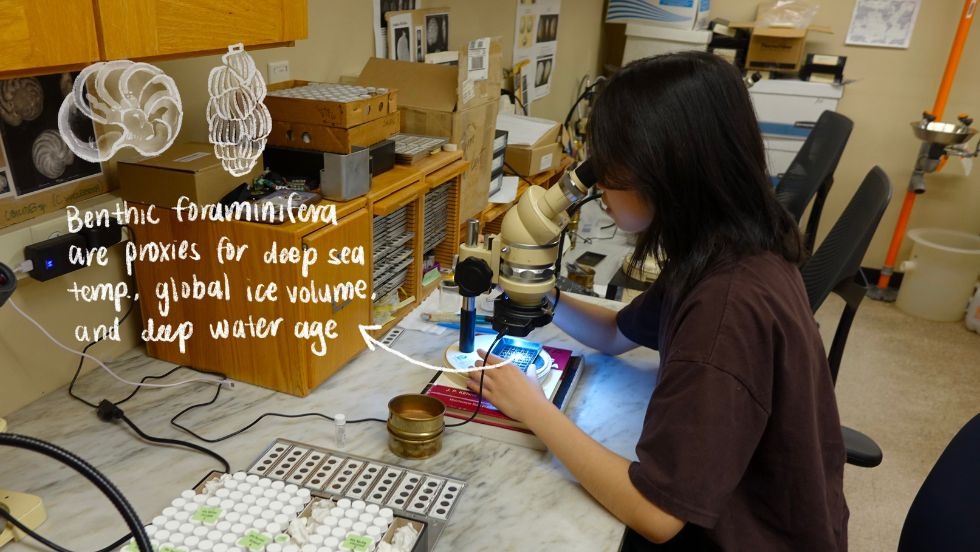
Claire Xu: "I am using benthic foraminifera from Site 846 in the eastern Equatorial Pacific to reconstruct δ18O and δ13C records for the Pliocene (5.3-2.6 million years ago)." (Advisor: Tim Herbert)
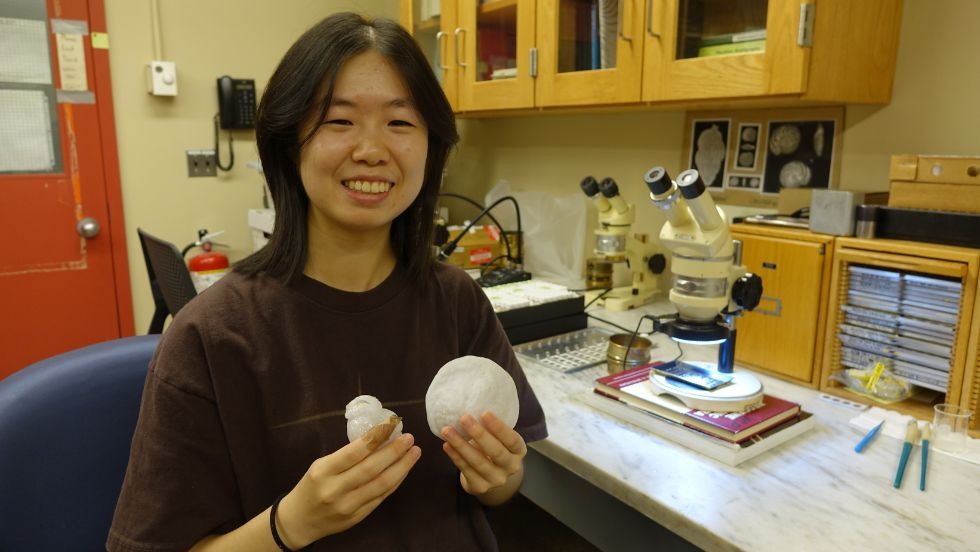
Claire Xu: "I am using benthic foraminifera from Site 846 in the eastern Equatorial Pacific to reconstruct δ18O and δ13C records for the Pliocene (5.3-2.6 million years ago)." (Advisor: Tim Herbert)

Andrea S. Jimenez: “I am using triple oxygen isotope measurements from Samoan lavas to constrain the origins of oxygen in the mantle and better understand the influence of crustal recycling in the Samoan mantle plume." (Advisor: James W. Dottin III, 2024 DEEPS-Leadership Alliance REU student from University of Puerto Rico at Mayagüez)
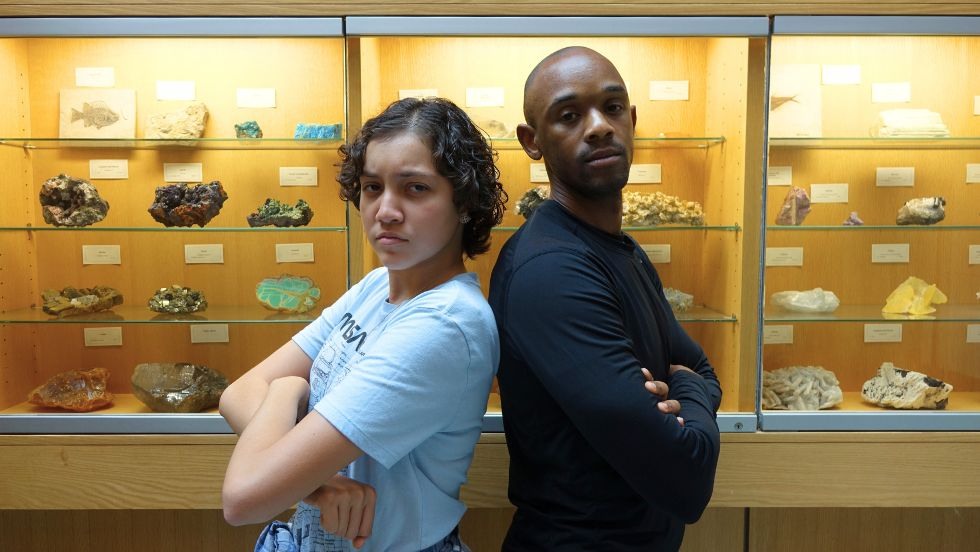
Andrea S. Jimenez: “I am using triple oxygen isotope measurements from Samoan lavas to constrain the origins of oxygen in the mantle and better understand the influence of crustal recycling in the Samoan mantle plume." (Advisor: James W. Dottin III, 2024 DEEPS-Leadership Alliance REU student from University of Puerto Rico at Mayagüez)
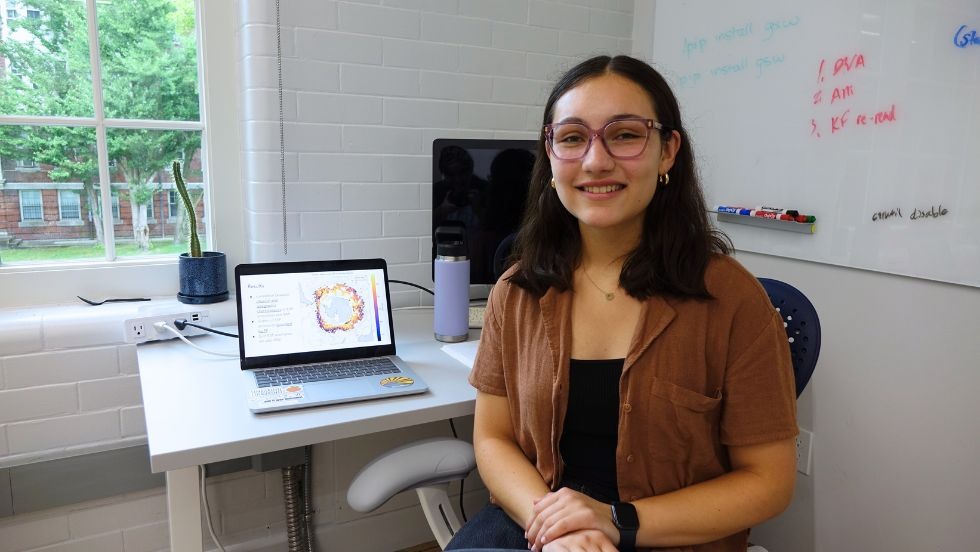
Margaret Gonzalez: “I am using observations from floats in the Southern Ocean to characterize Antarctic Winter Water (a cold water mass trapped below the surface Mixed Layer) and understand its connections to the carbon cycle." (Advisor: Mara Freilich, 2024 DEEPS-Leadership Alliance REU student from Loyola University Chicago)
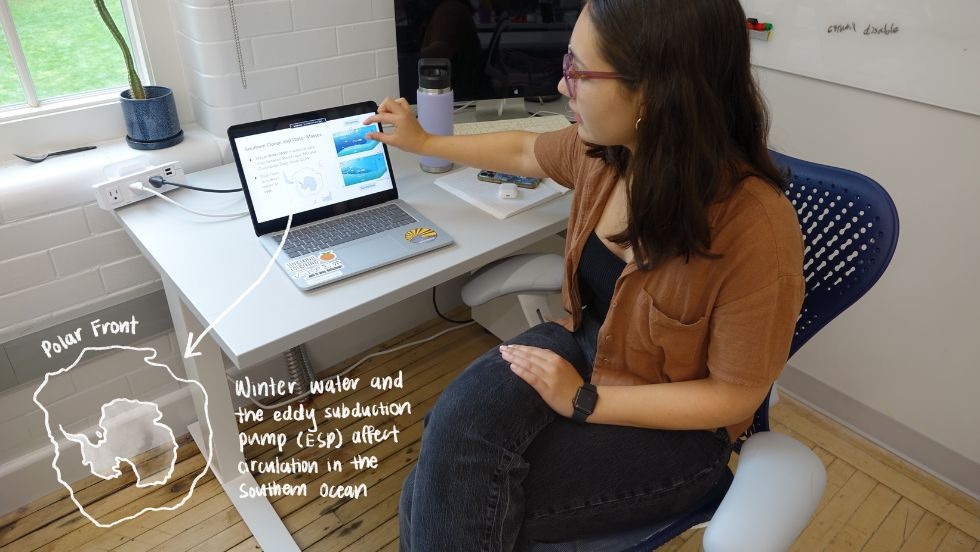
Margaret Gonzalez: “I am using observations from floats in the Southern Ocean to characterize Antarctic Winter Water (a cold water mass trapped below the surface Mixed Layer) and understand its connections to the carbon cycle." (Advisor: Mara Freilich, 2024 DEEPS-Leadership Alliance REU student from Loyola University Chicago)
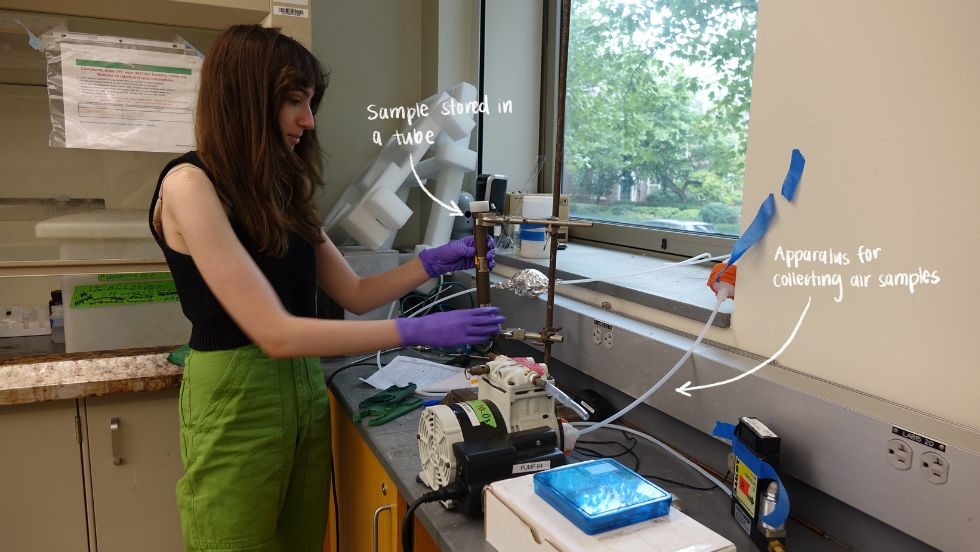
Emma Blankstein: “I am developing an ozone calibration for the Breathe Providence Project intra-urban air monitoring network and field collecting and analyzing ozone and NOx samples to better understand local sources and transport of pollution." (Advisor: Meredith Hastings)
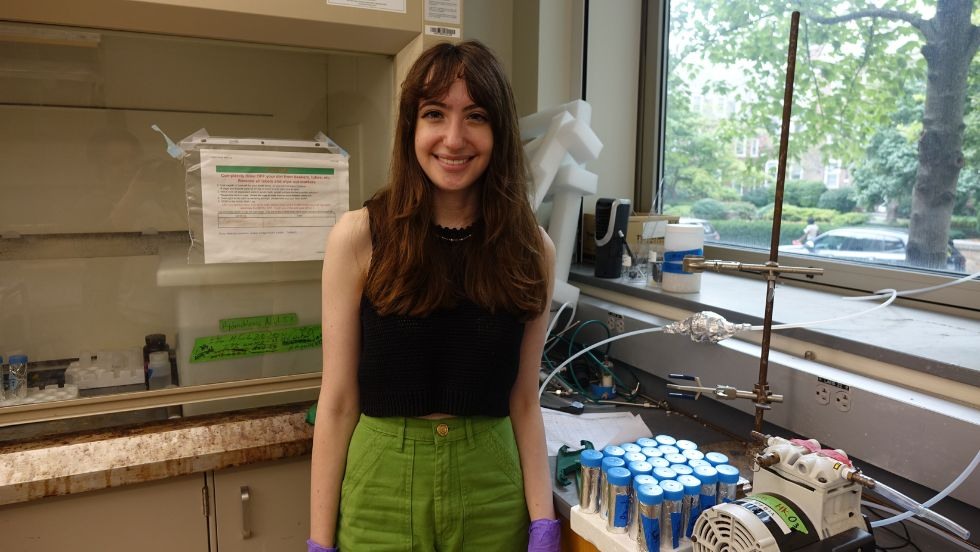
Emma Blankstein: “I am developing an ozone calibration for the Breathe Providence Project intra-urban air monitoring network and field collecting and analyzing ozone and NOx samples to better understand local sources and transport of pollution." (Advisor: Meredith Hastings)
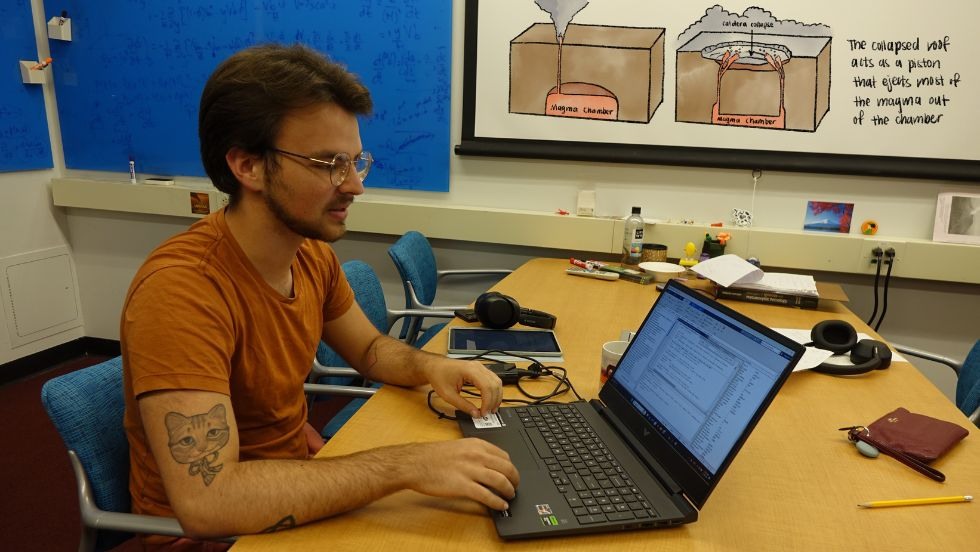
Gregorio Posada Pardo: “I'm using numerical modeling to identify the appropriate conditions required to replicate the magma chamber evolution of polycyclic caldera systems, with a focus on post-collapse recovery periods." (Advisor: Chris Huber)
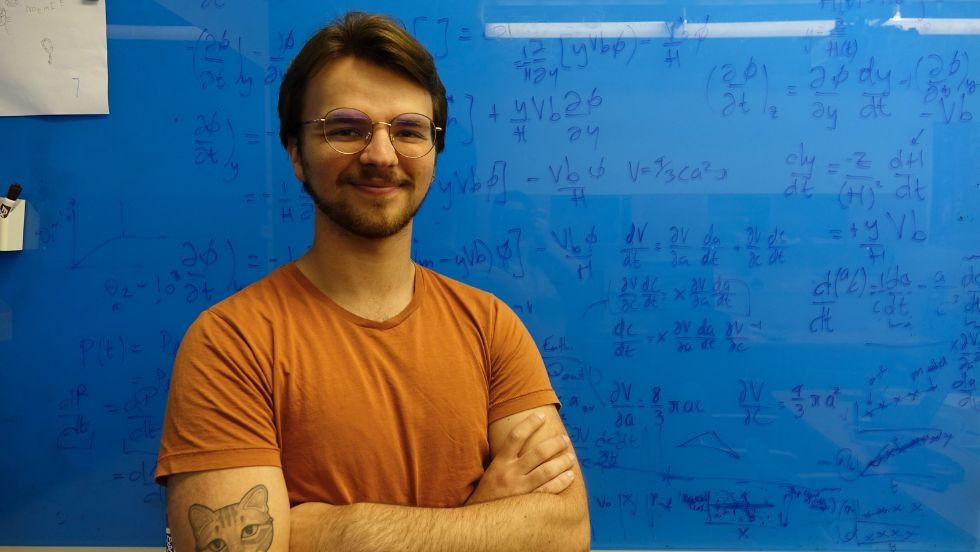
Gregorio Posada Pardo: “I'm using numerical modeling to identify the appropriate conditions required to replicate the magma chamber evolution of polycyclic caldera systems, with a focus on post-collapse recovery periods." (Advisor: Chris Huber)
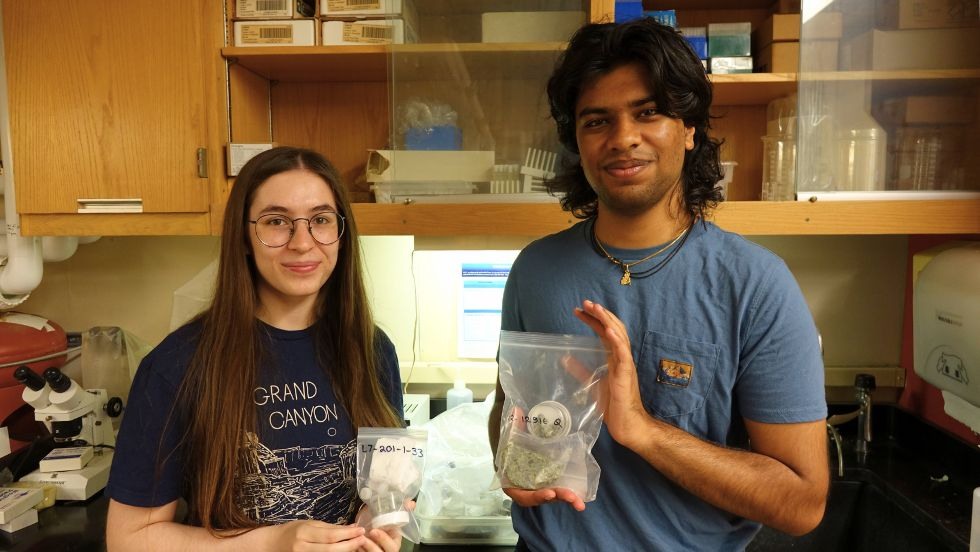
Shannon White and Dev Patel: “We are analyzing peridotite samples from Patagonia and Antarctica to attempt to model the mantle processes that formed them through inferences on bulk composition and dynamics." (Advisor: Alberto Saal)
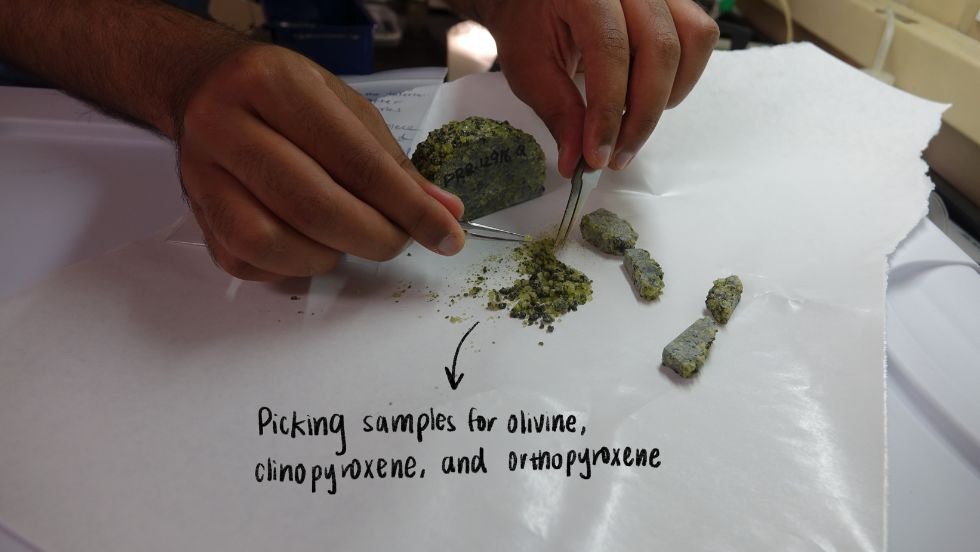
Shannon White and Dev Patel: “We are analyzing peridotite samples from Patagonia and Antarctica to attempt to model the mantle processes that formed them through inferences on bulk composition and dynamics." (Advisor: Alberto Saal)
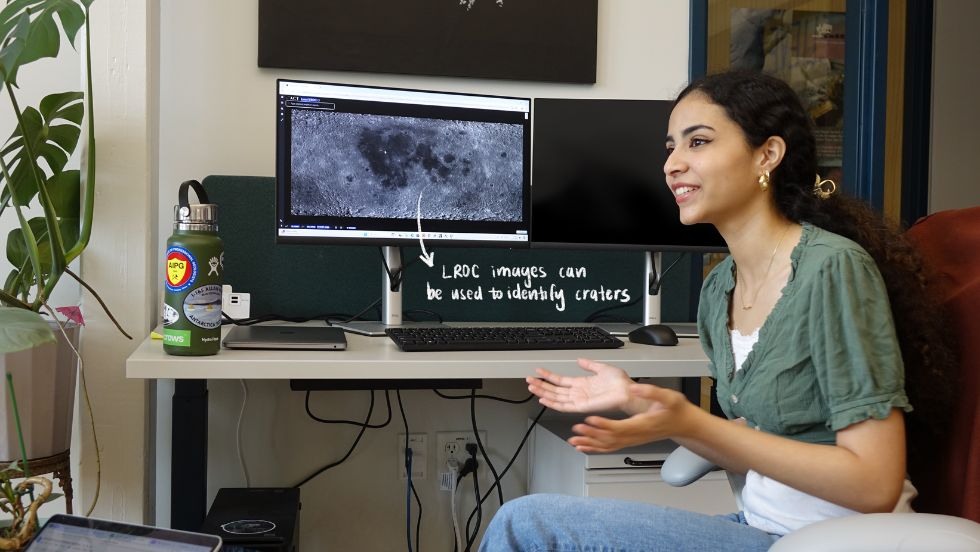
Sierra Khan: “I am locating small fresh craters on the Moon’s surface using LROC NAC pairs to search for the exact coordinates of known new impacts." (Advisor: Ingrid Daubar)

Sierra Khan: “I am locating small fresh craters on the Moon’s surface using LROC NAC pairs to search for the exact coordinates of known new impacts." (Advisor: Ingrid Daubar)
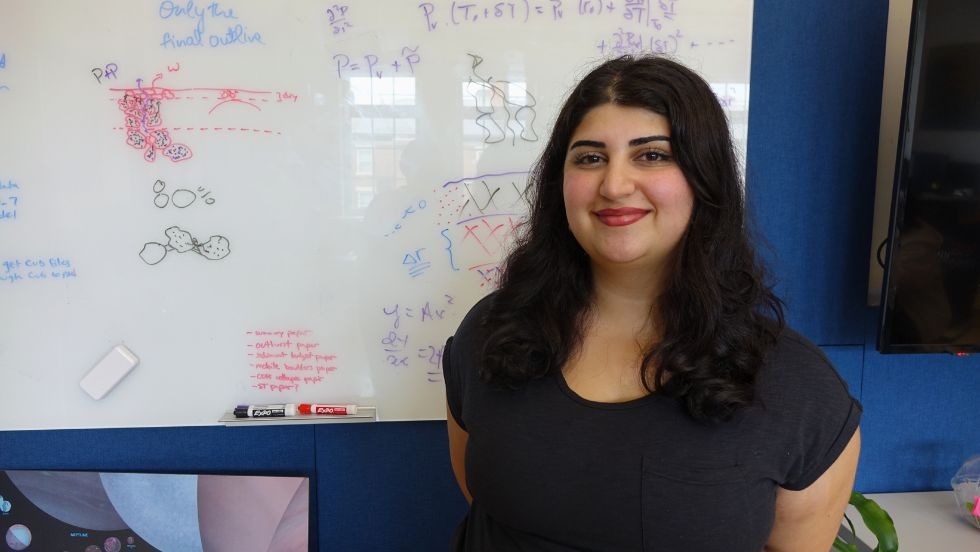
Ariyana Bonab: “I'm using HiRISE data of Mars to search for rockfalls on the planet’s surface in order to identify global triggers of Marsquakes and impact activity." (Advisor: Ingrid Daubar, Boston University)
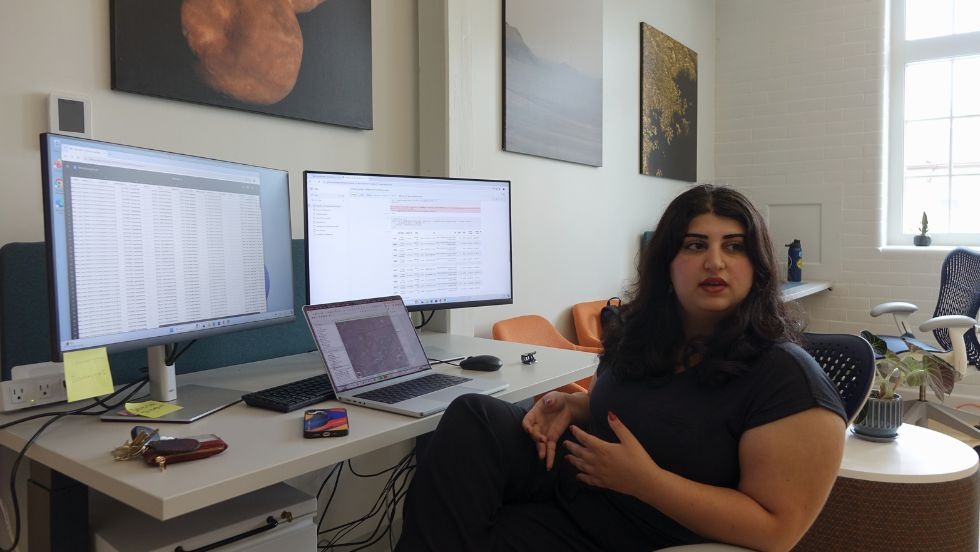
Ariyana Bonab: “I'm using HiRISE data of Mars to search for rockfalls on the planet’s surface in order to identify global triggers of Marsquakes and impact activity." (Advisor: Ingrid Daubar)
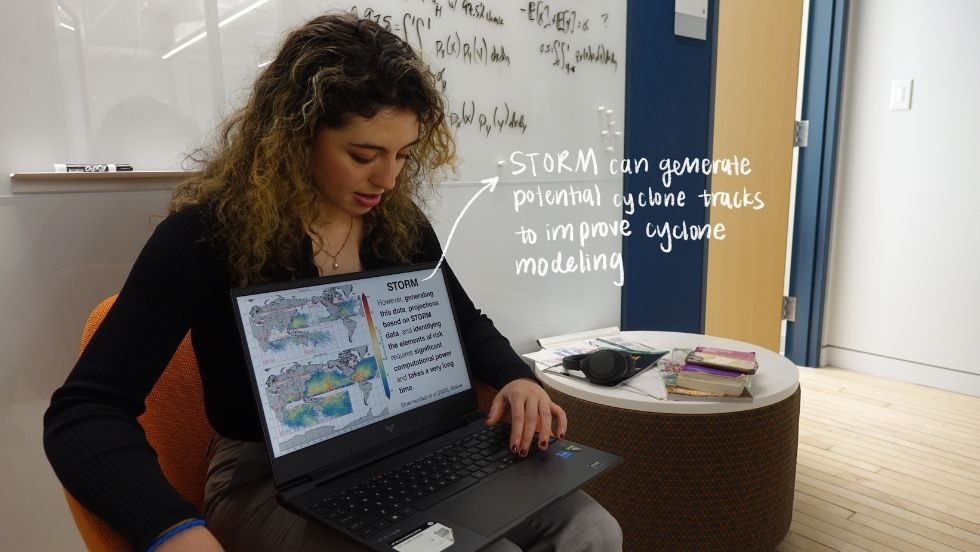
Abrielle Mannino: “I am using a U-Net model trained on STORM to create tropical cyclone predictions, and using this data to create maps visualizing the frequency of hurricanes in the South Pacific region and the likelihood they will threaten vital infrastructure for the UN South Pacific Community." (Advisor: Chris Horvat, 2024 DEEPS-Leadership Alliance REU student from Rollins College)
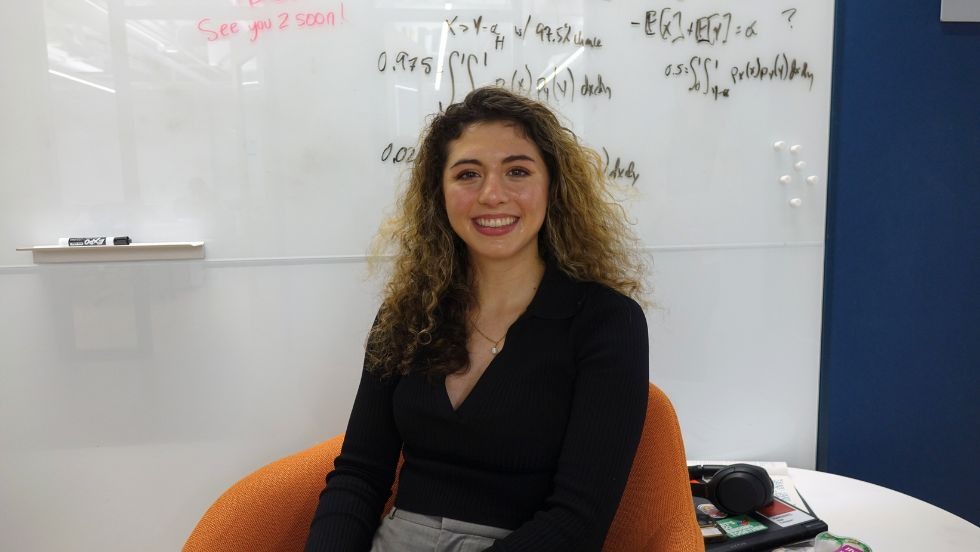
Abrielle Mannino: “I am using a U-Net model trained on STORM to create tropical cyclone predictions, and using this data to create maps visualizing the frequency of hurricanes in the South Pacific region and the likelihood they will threaten vital infrastructure for the UN South Pacific Community." (Advisor: Chris Horvat, 2024 DEEPS-Leadership Alliance REU student from Rollins College)
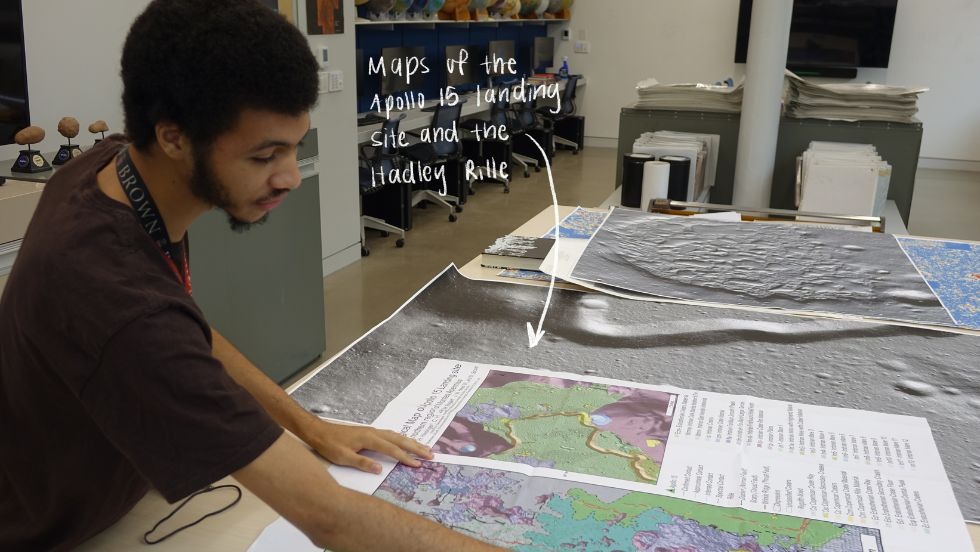
WaTae Mickey: “I'm revisiting the origin of the lunar sinuous rilles (specifically the Hadley Rille near the Apollo 15 landing site) using new topographic data and imagery in order to narrow down the origin of the Hadley Rille." (Advisor: Jim Head)
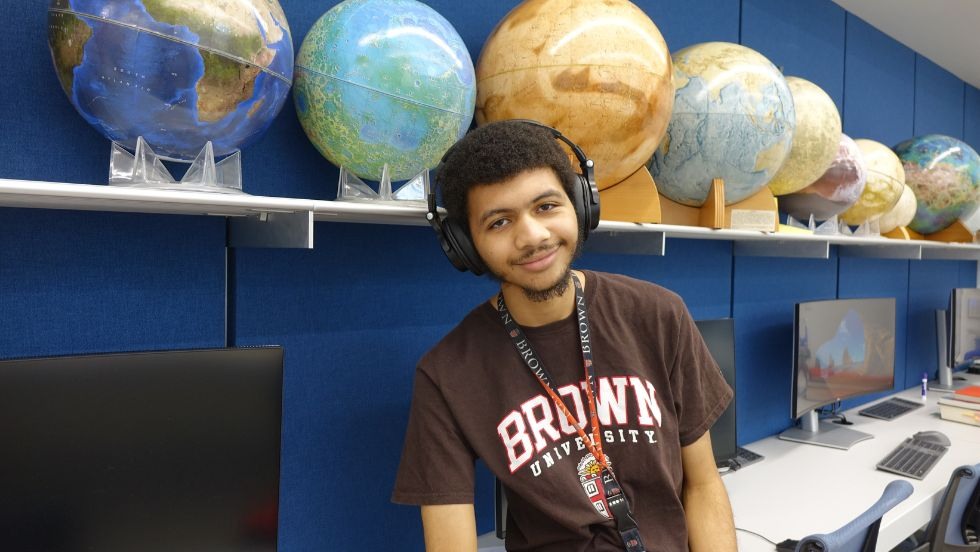
WaTae Mickey: “I'm revisiting the origin of the lunar sinuous rilles (specifically the Hadley Rille near the Apollo 15 landing site) using new topographic data and imagery in order to narrow down the origin of the Hadley Rille." (Advisor: Jim Head)
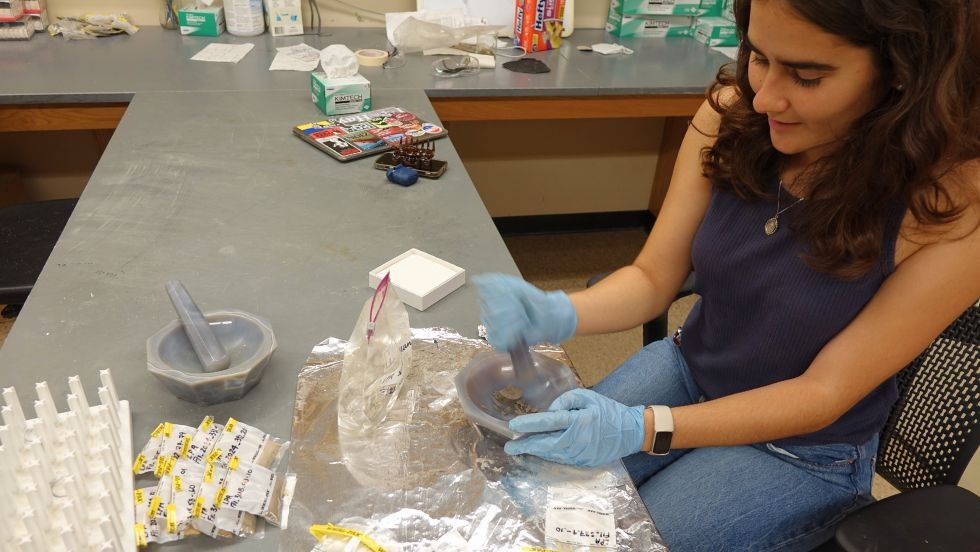
Isabel Tribe: "I am analyzing organic material preserved in a 600 meter deep terrestrial sediment core from Bogotá, Colombia to reconstruct the hydroclimate of the Northern Tropical Andes with a focus on the Pliocene, the last epoch during which temperatures and CO2 levels were higher than preindustrial levels." (Advisor: James Russell)
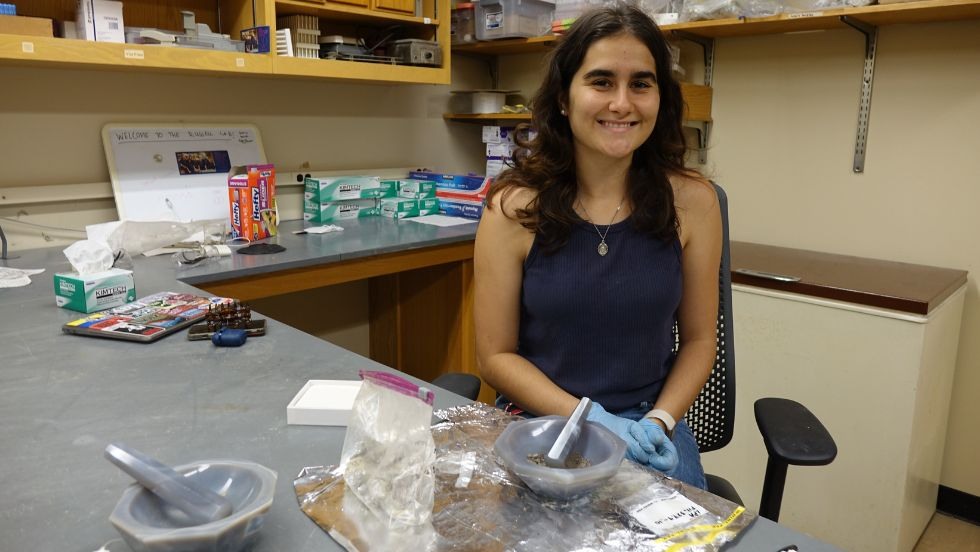
Isabel Tribe: "I am analyzing organic material preserved in a 600 meter deep terrestrial sediment core from Bogotá, Colombia to reconstruct the hydroclimate of the Northern Tropical Andes with a focus on the Pliocene, the last epoch during which temperatures and CO2 levels were higher than preindustrial levels." (Advisor: James Russell)
Read About Their Research
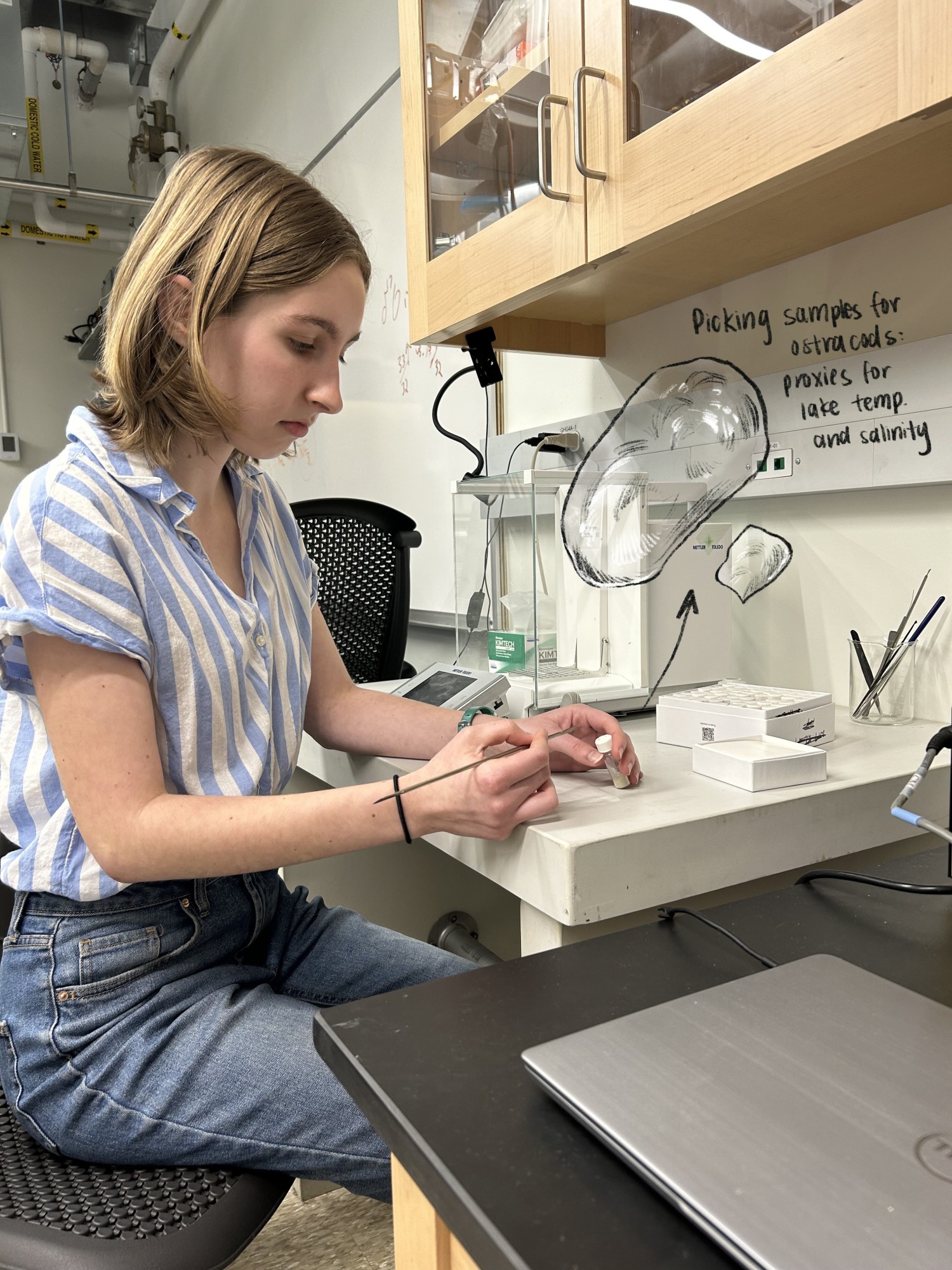
I am analyzing Western U.S. ostracod fossils from Silver Lake, historically Glacial Lake Mojave, to assess the lake’s conditions across the last deglaciation. Ostracods are microscopic aquatic crustaceans that serve as paleoclimate proxies for lake conditions such as temperature and salinity. My work involves identifying and cataloging ostracod species under the microscope, and the species data can indicate specific lake characteristics, because different species tolerate different ranges of conditions. I also prepare samples for ICP-MS, a technique that uses plasma to excite and measure trace elements like Mg/Ca and Sr/Ca, both proxies for salinity. By collecting species data and analyzing the elemental and isotopic composition of the ostracods, we can reconstruct the conditions of Glacial Lake Mojave between 24-10 kya and potentially use our data to model the area’s climate over that period.
It’s exciting how the record from the ostracods aligns nicely with that from a carbonate rock called tufa, another proxy for lake conditions. The correlation between the isotope signals in both records substantiates our theories about past lake conditions. I’ve especially enjoyed the species identification work— I find it cool figuring out the different types of ostracod species present in the samples, making comparisons, and applying an ecological lens to a geoscience project.
Advisor: Dan Ibarra
I am creating a time series using stable isotope analysis of tufa records from Glacial Lake Mojave (also known as Silver Lake, located near modern day Los Angeles, CA) to assess changes in lake conditions across the last deglacial period. This area is important for understanding how water availability fluctuates in response to climate change, as the region previously had lakes that have since dried up during the last deglaciation.
Water ability in this region depends on atmospheric rivers, or jet streams, that deliver precipitation to the Western US.However, precipitation projections differ between climate models. My work, which involves samples of tufa (carbonate rock) for d13C and d18O isotopic analyses, generates a new record for the southern US that gives insight into shoreline levels and the forcings that affected water availability since the last glacial maximum. This record contributes to existing studies of this southern region and provides more data to refine climate models. So far, the tufa data aligns well with ostracod data from the same location.
I was drawn to this research because of its practical applications: we want to understand how water supply is affected by climatic processes and climate change so we can manage future water availability in arid regions. Additionally, the most exciting part of being in the REU program was not only doing research but getting to know my peers’ work across multiple fields. Being able to share our experiences is very fulfilling and mind-opening, and it’s exciting to know there’s so much more out there to discover.
2024 DEEPS-Leadership Alliance REU student from Dallas College
Advisor: Dan Ibarra
I am using benthic foraminiferal fossils of the Milioda order to uncover the extent of methane hydrate dissociation and subsequent methane release in the Indian Ocean over the past 1.4 million years. In the Sulfate-Methane Transition Zone (SMTZ), located beneath the seafloor, bacteria consume sulfate and methane to produce bicarbonate, whose carbon signature is taken up by the forams when they form their calcium carbonate shells. Methane, a biological product, tends to contain more 12C instead of 13C because biological processes, like photosynthesis, favor the lighter 12C isotope over the heavier 13C isotope. So, the generated bicarbonate used by forams—and thus, forams themselves—have a low δ13C signature. More negative δ13C values indicate a larger release of methane and therefore greater methane hydrate dissociation.
We’re interested in generating this timeline because there are currently no continuous records of methane hydrate dissociation along the continental shelf and rise of the Indian Ocean. Therefore, examining samples from the past 1.4 million years (a period that captures numerous glacial-interglacial cycles) allows us to look at how the carbonate isotope signature has changed over time and determine whether the cycles correlate with methane releases. My work involves picking and cleaning forams for mass spectrometry, and it’s really cool to contribute to a project that creates new knowledge. I’ve also greatly enjoyed the camaraderie in the department this summer.
Advisor: Steve Clemens
Reconstructing Earth's past climate can give insight into the long-term effects of anthropogenic global warming. One proxy used in climate reconstructions is benthic foraminifera: single-cell microorganisms living in the deep ocean that preserve the oxygen (δ18O) and carbon isotope (δ13C) ratios of surrounding water in their calcium carbonate shells. These values correlate with climate characteristics such as deep sea temperature, global ice volume, and deep-water age on thousand-year timescales.
I am using benthic foraminifera from Site 846 in the eastern Equatorial Pacific to reconstruct δ18O and δ13C records for the Pliocene (5.3-2.6 million years ago). This period is especially relevant for climate projections since simulations indicate the Pliocene as a likely analog of global climate in 2030. This real-world application of my work is fascinating to me: we aim to understand not only how Earth’s climate evolved in the past, but how it may progress in response to global warming in the future. Notably, the Pliocene had similar carbon dioxide levels to today but no permanent Northern Hemisphere ice sheet, higher sea levels, and warmer global temperatures.
Besides gaining insight into university-level research, I greatly enjoyed putting together this year’s Summer Research Highlights. The opportunity to discuss topics my peers were passionate about, draw connections between our research projects, and share our summer adventures was extremely fulfilling. I am blown away by their knowledge, and grateful to have made new friends! I also loved that I could combine my interests in Earth science and scientific illustration.
Advisor: Tim Herbert
I am using triple oxygen isotope measurements from Samoan lavas to constrain the origins of oxygen in the mantle and better understand the influence of crustal recycling in the Samoan mantle plume. Previous research shows that lavas from the different south-central Pacific islands display distinct sulfur compositions. My work, which involves using laser fluorination and mass spectrometry to obtain isotopic measurements of the samples, aims to investigate whether the islands also have distinct oxygen compositions. We found that oxygen compositions are equal throughout all the islands; since oxygen is so widespread, there is a possibility that it was homogenized in the mantle. There is also a possibility that the plume is homogenous but the plate is heterogeneous, unlike previously believed.
The overarching goal of this project is to better understand crustal recycling and the flux in the amount of oxygen in the crust, since subduction of the mantle has a major role in controlling the oxygen budget at Earth’s surface. We can also gain insight into how mantle subduction affects the eruption of volcanoes and the formation of islands.
My main interest is planetary science, but working in geochemistry has allowed me to deepen my understanding of Earth processes and build knowledge to apply these concepts to other planets in future work. Through this opportunity, I’ve also met so many people, from my DEEPS Leadership Alliance cohort to faculty in the department, and it's been wonderful getting to know their wide ranges of interests and disciplines.
2024 DEEPS-Leadership Alliance REU student from University of Puerto Rico at Mayagüez
Advisor: James W. Dottin III
I am using observations from floats in the Southern Ocean to characterize Antarctic Winter Water (a cold water mass trapped below the surface Mixed Layer) and understand its connections to the carbon cycle. My work involves analyzing and visualizing the float data to identify locations of Winter Water and the eddy subduction pump (ESP). Winter Water can be identified by temperature decreases at certain depths; ESP anomalies, small circulation events that pull down surface water, can be identified from spice (a temperature and salinity variable that distinguishes water masses) and AOU (oxygen levels that indicates the time since the water in question was at the surface) decreases at certain depths. When all three decreases are present, an ESP anomaly is identified as Winter Water.
Since ESP is part of the biological pump and draws down carbon through the subduction of water, identifying ESP anomalies as Winter Water allows us to determine how Winter Water affects the carbon cycle. This improved understanding of carbon cycle influences will help with developing more successful adaptation solutions to climate change, since we can refine our understanding of the climate system to better reflect ocean conditions. Throughout this project, I’ve particularly enjoyed making visually pleasing and engaging graphs in Python, and being able to present science in a way that is accessible to the public.
2024 DEEPS-Leadership Alliance REU student from Loyola University Chicago
Advisor: Mara Freilich
I am developing an ozone calibration for the Breathe Providence Project intra-urban air monitoring network and field collecting and analyzing ozone and NOx samples to better understand local sources and transport of pollution.
I am also creating a mathematical model that aims to identify a mathematical relationship between the Breath Providence and EPA sensors. Since EPA monitors are sparse and cannot comprehensively detect pollutants, we hope to calibrate the Breathe Providence sensors to the EPA ones so we can learn more about the diurnal and annual patterns in pollutant source and transport. For instance, we see a correlation between high heat and high ozone levels, which we can use to develop warning systems and ozone layer predictions to help people better protect themselves.
I’ve really enjoyed collecting the samples and doing isotopic composition analyses in the lab. Moreover, as Breathe Providence is community-driven and responsive to community concerns and needs, I’ve valued being able to use theoretical concepts about pollution for good through a project with immediate impact for real people.
Advisor: Meredith Hastings
I'm using numerical modeling to identify the appropriate conditions required to replicate the magma chamber evolution of polycyclic caldera systems, with a focus on post-collapse recovery periods. Caldera systems have massive ellipsoidal magma chambers that grow wide enough for the roof above them to become unstable and collapse, resulting in an eruption that can eject almost all of the magma in the chamber, forming a caldera in the process. Some of these caldera systems go through a “caldera cycle,” where after maturation and a subsequent period of fermentation, the chamber roof collapses again.
My work involves adding onto an already existing magma chamber box model developed by Huber and Degruyter in order to make it applicable for polycyclic caldera systems. I’ve been involved in adding the trigger criteria for caldera eruptions, and setting up the relevant modifications for the chamber to grow horizontally into an ellipsoid as opposed to radially into a sphere. With these new processes being added to the model, we are looking at how the timescales of magma injection, chamber cooling, and viscous relaxation of the crust define the conditions needed for these systems to become cyclic, and whether we can use these ranges of conditions to improve our predictions on when a system will experience roof collapse again.
This project allowed me to learn more about coding, physical volcanology, and numerical modeling through hands-on research. By the end of the Summer, with guidance from my PI and lab mates, I was able to confidently navigate the code I was given, and utilize the concepts I had learned about the dynamics of magma chambers to actively build towards our goal. I also enjoyed getting to know the research environment from an inside perspective, and experiencing how people collaborate and bounce ideas off of each other to work on developing tools for solving pressing questions in the field of geoscience.
Advisor: Chris Huber
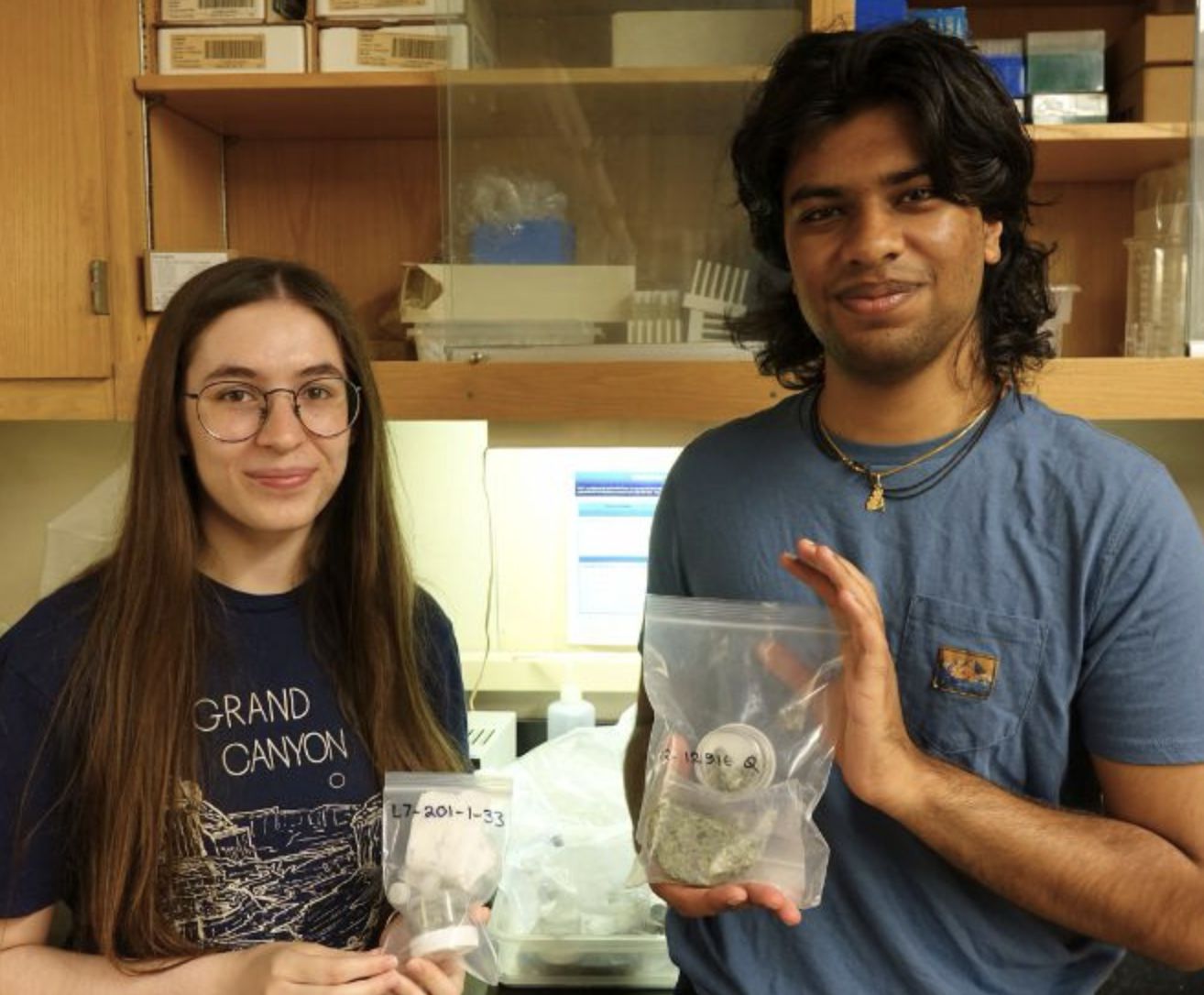
We are analyzing peridotite samples from Patagonia and Antarctica to attempt to model the mantle processes that formed them through inferences on bulk composition and dynamics.
This summer, we established strong lab techniques for preparing samples for instrument analysis. Our work consisted of making sections in the rock, cutting out cores, and picking out grains of olivine, clinopyroxene, and orthopyroxene into multiple size fractions which will later undergo laser ablation. Laser ablation will indicate the rocks’ compositions, and potentially the temperatures at which they formed. This coming semester, we will be applying analytical techniques to the data we collect from instrument analysis. Since these rock samples come from the mantle, their compositional characteristics—such as the percentage of trace elements present—can tell us how significantly they’ve been altered. Using this data, we will determine if these rocks are an accurate representation of the mantle.
Tracking the trace elements and using laser ablations was really cool, as we got to see the individual grains of our samples and start to determine if they’ve been altered. Such small samples can have such a large impact on our understanding of the mantle. It was also interesting learning how mindful we had to be in generating samples, since we only had a certain amount to work with. It was really valuable to have this research opportunity available to us even as we were also taking summer courses during this project.
Advisor: Alberto Saal
I am locating small fresh craters on the Moon’s surface using LROC NAC pairs to search for the exact coordinates of known new impacts. The Lunar Reconnaissance Orbiter Camera (LROC) narrow-angle camera (NAC) captured images of the Moon starting in 2009. By overlaying temporal pairs—two photos of the same location taken at different times with an incidence difference of no more than 3 degrees—I can find new craters that have formed. The goal of this project is to create a more comprehensive dataset of moon craters and to better understand the properties of the lunar surface. So far, 25 of the approximately 222 craters have been identified.
During this project, I was surprised and excited to find impact splotches; these are recent changes in the surface's albedo, which are most likely of impact origin and possibly harbor previously unknown craters with diameters smaller than 1 meter.
Advisor: Ingrid Daubar
I am using a U-Net model trained on STORM to create tropical cyclone predictions, and using this data to create maps visualizing the frequency of hurricanes in the South Pacific region and the likelihood they will threaten vital infrastructure for the UN South Pacific Community. Previously, cyclone tracks have not been able to be modeled comprehensively due to limited data. By utilizing machine learning through STORM, a cyclone track model, to resample and generate more tracks, the U-Net model can create a higher resolution image of potential cyclone landfalls. However, these physics-based models typically produce outputs that are not human-relevant, making it difficult to communicate results to government agencies and policymakers in affected areas. Therefore, I’m also mapping hurricane frequency for various Pacific Island countries and territories such as Vanuatu, Fiji, and Solomon islands, all places that are the first and worst impacted by climate change.
Through this project, a joint initiative with the UN, we hope to provide useful information that stakeholders can use to strengthen tropical cyclone resilience in their communities with efforts like expanding healthcare facilities and improving infrastructure. Future goals include running the models with new conditions and parameters to simulate a broader range of potential situations, providing this information to policymakers, and adding individual risk assessments for hospitals. I’ve valued having this opportunity to talk about topics important to me, such as climate change and natural disaster resilience. I also enjoyed building connections within DEEPS, who were friendly and welcoming during my time at Brown this summer.
2024 DEEPS-Leadership Alliance REU student from Rollins College
Advisor: Chris Horvat
I'm using HiRISE data of Mars to search for rockfalls on the planet’s surface in order to identify global triggers of Marsquakes and impact activity. By examining these high-res images through a convolutional neural network trained on Mars rockfall data, we can map the location of rockfalls to determine terrain characteristics like bearing capacity, mass wasting processes, and energy estimates of impact activity. This research will then be used for global bearing capacity calculations for the ExoMars/Rosalind Franklin rover landing site in 2028 to ensure safe landing in the geologically active region of Oxia Planum.
By identifying rockfall candidates, we’re also identifying the false positives picked up by the convolutional neural network, where geomorphological features are labeled as rockfalls but are actually something else, like gullies or polygonial terrain. We are also measuring the changes in the surface over short timescales by re-requesting HiRISE images to be taken with the Mars rover. By doing this, we will determine if there has been recent geological activity in the region. Alterations to Mars’ surface can be identified through change detection: comparing images from 2004-2006 to re-requested images taken 18-20 years later.
I’ve been working on this project for over a year, and one of my most memorable experiences has been attending the LPSC conference, where I got to meet so many amazing people from NASA and DEEPS. I enjoyed presenting to such a receptive and caring audience—people will ask questions about your work because they’re as curious and passionate as you are. My experience at DEEPS solidified my desire to pursue research post-grad. I never want to stop being curious about our universe.
Boston University
Advisor: Ingrid Daubar
I'm revisiting the origin of the lunar sinuous rilles (specifically the Hadley Rille near the Apollo 15 landing site) using new topographic data and imagery in order to narrow down the origin of the Hadley Rille.
Sinuous rilles, or channels on the Moon’s surface, are agreed to have volcanic origins, but the specific mechanisms behind their formation are not agreed upon. There are five proposed hypotheses for rille formation: filled faults, thermal erosion, surface lava channel formation, lava tube collapse, and lava basin drainage. The mechanisms forming the rilles may be a combination of all of these factors; however, drawing such conclusions requires good resolution imagery and physical samples of the rille. Thus, exploration of the Hadley Rille is tied to further human and robotic exploration of the lunar surface.
The Hadley Max 500 day mission is a design reference mission my research group is planning in order to revisit the Apollo 15 landing site. Should this new mission happen, the landing site will be right next to the Apollo 15 landing site, allowing convict access to Hadley Rille and would serve as crucial experience needed for future missions to other planets.
An unforgettable moment from working on this project was being on call with the team when they were informed about winning NASA's Innovative and Advanced Concepts (NIAC) Phase III and seeing their candid enthusiasm. I’ve also enjoyed the opportunity to begin writing a paper and gain experience with scientific writing, as working with researchers who major in a wide variety of subjects.
Advisor: Jim Head
I am analyzing organic material preserved in a 600 meter deep terrestrial sediment core from Bogotá, Colombia to reconstruct the hydroclimate of the Northern Tropical Andes with a focus on the Pliocene, the last epoch during which temperatures and CO2 levels were higher than preindustrial levels. During the last 5 million years, the Bogotá basin has experienced transgressions and regressions, going from dry to lake to swamp conditions. My work includes crushing samples with a mortar and pestle, running samples through the accelerated solvent extractor (ASE) to extract the lipids, and isolating biomarkers with different chemicals through column chromatography. The lipids from leaf waxes in the sediment core—n-alkanes and branched GDGTs—serve as proxies for hydrology and temperature, respectively.
The goal of my research is to better understand how the hydroclimate of the Northern Tropical Andes is affected by human-induced climate change. Tropical latitudes are largely understudied, even though they are home to 40% of the world’s population, the majority of whom live in low-income countries that are under-equipped to handle the effects of climate change. We hope to provide an accurate hindcast dataset that can be used to train climate models and ultimately improve long-term climate projections, helping these communities better prepare for the future in a warming world.
Working in a female-dominated lab and seeing women at the helm of groundbreaking research is really inspiring. My supervisor, postdoctoral research associate Lina Pérez-Angel, is Colombian, and it’s empowering to see her study her own country in a majority white, male, and anglo-centric field.
Advisor: James Russell